OVERVIEW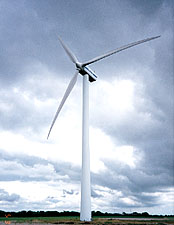
This article is based on power system simulation studies carried out by VTT Processes of Finland (a division of VTT Technical Research Centre of Finland that conducts research in the area of bioenergy and distributed energy), and the University of Vaasa. The PSCAD (Power System Computer-Aided-Design) power system simulation software developed by the Manitoba HVDC Research Centre has been applied to these studies. One of the key issues has been to verify that traditional network protection schemes and settings are simply not adequate when there are Distributed Generation (DG) systems connected to the network. The protection coordination between network protection and the protection of the DG units has also been studied. The results of these studies will be used to focus further research and development in protection systems and concepts.
WIND ENERGY INTERCONNECTION
As a renewable energy source, wind turbines are gaining great popularity. They are relatively low in capital cost, and can be brought into service relatively quickly. It is not surprising then that investors are looking at large wind farms with power ratings of the order of hundreds of MW, and in some cases, simulation studies are investigating capacities of thousands of MW of Wind Energy. In addition to large wind farms connected to transmission networks, smaller wind farms and individual wind power plants connected to distribution networks, are being built.
The protection and control requirements of wind power systems are different in transmission networks and distribution networks. Transmission network operators are interested in power system stability issues. There are set requirements that must be met related to the control of active power, voltage and frequency. In network fault situations, fault tolerance may be required in order to avoid major disturbances due to common mode tripping. In distribution networks however, the requirements are often quite different, e.g. instead of fault tolerance, rapid disconnection of DG is required in the event of network faults.
To be able to maintain power quality and reliability of a distribution network or a transmission network, the impact of wind power must be carefully studied. The interaction between a power plant and the power system is typically verified by means of power system simulations. In order to make these simulations possible, wind farm owners are required to provide system operators with the complete system models. Dynamic simulation using accurate system models is an indispensable tool both in transmission and distribution level interconnection studies.
THE IMPACT OF DISTRIBUTED GENERATION
Traditionally distribution networks have been designed to operate radially so that the power flows from upper voltage levels down to customers situated along the radial feeders. This has enabled a relatively straightforward protection strategy. When applying over-current protection, for example, it has been possible to assume that the fault current can have only one direction. However, this is now not always true if there are distributed generation (DG) units such as wind turbines in the network! As the share of distributed generation increases, distribution networks are becoming more like transmission networks where generation and load nodes are mixed, and a more complex protection system design is unavoidable.
In order to analyze the effects of distributed generation on the requirements for the protection of distribution networks, power system simulation studies are required. The dynamic modeling of various types of DG technologies such as wind energy is a necessity.
POTENTIAL PROBLEMS TO PROTECTION
Power system simulation and modeling studies have shown that distributed generation causes several challenges to the protection of distribution networks. The most commonly mentioned problems are the following:
The appearance of these kinds of problems depends on both the characteristics of the network and DG. E.g., in short-circuit faults a wind power plant generates fault current that depends strongly both on the wind turbine generator type and the network configuration. Synchronous generators are able to feed rather large sustained fault current while inverter based systems may be controlled so that their output may be limited even to the rated current. From the point of view of protection coordination, the location of the fault in relation to generator and protection devices dictates the outcome of the fault situation.
Some critical problems related to DG are evaluated:
THE BLINDING OF PROTECTION - PREVENTING OVERCURRENT RELAY OPERATION
When a large distributed generation production unit or several small ones are connected to a medium voltage network, the fault current seen by the feeder protection relay may be reduced, which can lead to the prevention of the operation of overcurrent relays. This is also called protection under-reach.
Theoretically the problem can be described as follows. A situation, where a production generator unit is connected in a medium voltage (MV) feeder close to a primary substation, is studied. When a fault occurs at the end of the feeder the fault current consists of contributions both from the grid (I1) and from the generator (I2) as shown in Figure 1. The impedances involved in this situation are:
Zs = impedance of the grid and the primary transformer
Zg = impedance of the generator
ZL = impedance of the feeder (line)
The current contribution from the distributed generator reduces the current seen by the feeder relay. We can conclude that the impact of the production unit increases with the size of the generator and with the length of the line section between the production unit and the fault.
Simulation Results
Figure 3 presents power system simulation results of the current seen by the feeder overcurrent relay, when a large (8 MW) synchronous generator (an example of distributed generation) is connected to different locations (0 km, 12 km, 20 km and 34 km) on the feeder, and a three-phase fault occurs at the end of the feeder. The relay pickup current is 300 A, and the time delay is 0.5 s. The fault current without the power plant would be ca. 420 A. The fault occurs at time 10.1 s. In three of the above mentioned cases, the power plant reduces the current seen by the protection so much that the relay will not start until the power plant trips, which in these cases takes several seconds.
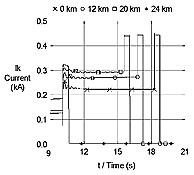
Figure 3. Current seen by the feeder overcurrent relay when the distance of a distributed power source from the substation is varied.
FALSE TRIPPINGS
The basic principle of false tripping is shown in Figure 4. The short-circuit fault occurs on feeder 2, but also feeder 1 is tripped because of overcurrent fed by the DG unit. False tripping (sympathetic tripping) is typically caused by synchronous generators, which are capable of feeding sustained short-circuit current.
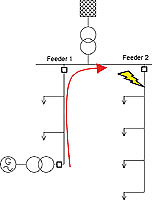
Figure 4. Principle of false tripping due to Distributed Generation.
In our study, false tripping of a healthy feeder system was verified by simulations with the 8 MW synchronous generator model. Figure 5 presents an example where a 3-phase short-circuit fault on an adjacent feeder causes the false tripping of feeder 1, because the power plant on feeder 1 feeds short-circuit current to the fault.

Figure 5. Example of false tripping caused by a short-circuit fault on adjacent feeder.
False tripping of healthy feeders can likely be solved by directional overcurrent relays, but with the following consideration: Protection against the bus faults may have to be changed. There should be a transfer trip from the main infeed relays (overcurrent relays on the secondary side of the primary transformer) and the arc protection relays to the feeder relays for the feeders having a significant amount of DG connected.
ANTI-ISLANDING PROTECTION
In the case of a sudden loss of grid connection, a part of the network may keep operating as an island. In most cases this is not desirable for the following reasons: • Reconnection of the islanded part becomes complicated, especially when automatic reclosing is used. This can lead to damage of equipment and can decrease the reliability of the network.
In terms of achieving an adequate safety and reliability level in the distribution system, Anti-islanding protection is generally considered necessary. The rules and guidelines vary from country to country but requirements similar to the following are often given: • DG should be disconnected from the network in the case of an abnormality in voltage or frequency.
Anti-islanding protection is very hard to accomplish by traditional means, e.g., the basic under-/over-voltage and under-/over-frequency relays may fail to operate if the power mismatch in the island that is created in the islanding situation is close to zero.
AUTORECLOSING
In overhead medium voltage networks, automatic reclosing is a very effective means to clear faults. In Finland for example, approximately 80% of faults can be cleared with high-speed autoreclosing and 15% with time-delayed autoreclosing. The impact of autoreclosing is based on the extinction of arc during the dead time of the reclosing sequence. In the Nordic countries of Europe, dead time is typically only 0.3 s.
Distributed generation seems to be rather incompatible with present reclosing practices. In suitable conditions, DG may prevent the arc extinction and the momentary fault can become a permanent fault. During the circuit breaker open time of the reclosing sequence, the generators in the network usually tend to drift away from the synchronism with respect to the grid. Thus the reconnection made without any synchronization, which is the usual way, may cause serious damages to the distributed generators, as well as high currents and voltages in the neighboring network.
Power system simulation studies have confirmed the high risk of out-of-phase reclosing. It can be safely stated that rapid and reliable loss-of-mains protection is needed. Distributed Generation units must be disconnected very rapidly during the breaker open time of an auto-reclosing sequence.
SUMMARY
According to the simulation studies performed with PSCAD, the proper coordination of the protection of network and distributed generation units is highly important in order to avoid false tripping of protection devices. In certain cases directional protection is also necessary. And, DG units that are capable of providing large short-circuit current may prevent the operation of feeder relays.
The primary results of these simulation studies have provided a clear indication of the potential protection problems that need to be solved by careful protection design or possibly through the introduction of new protection algorithms. Solutions are urgently needed since the percentage of distributed generation is increasing rapidly.
ACKNOWLEDGEMENT
The authors would like to acknowledge the insight and contributions of the following individual:
Dennis Woodford, President, Electranix Corporation for his contribution on the dynamic modeling of wind energy systems.
ABOUT THE AUTHORS
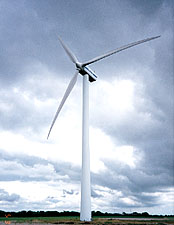
This article is based on power system simulation studies carried out by VTT Processes of Finland (a division of VTT Technical Research Centre of Finland that conducts research in the area of bioenergy and distributed energy), and the University of Vaasa. The PSCAD (Power System Computer-Aided-Design) power system simulation software developed by the Manitoba HVDC Research Centre has been applied to these studies. One of the key issues has been to verify that traditional network protection schemes and settings are simply not adequate when there are Distributed Generation (DG) systems connected to the network. The protection coordination between network protection and the protection of the DG units has also been studied. The results of these studies will be used to focus further research and development in protection systems and concepts.
WIND ENERGY INTERCONNECTION
As a renewable energy source, wind turbines are gaining great popularity. They are relatively low in capital cost, and can be brought into service relatively quickly. It is not surprising then that investors are looking at large wind farms with power ratings of the order of hundreds of MW, and in some cases, simulation studies are investigating capacities of thousands of MW of Wind Energy. In addition to large wind farms connected to transmission networks, smaller wind farms and individual wind power plants connected to distribution networks, are being built.
The protection and control requirements of wind power systems are different in transmission networks and distribution networks. Transmission network operators are interested in power system stability issues. There are set requirements that must be met related to the control of active power, voltage and frequency. In network fault situations, fault tolerance may be required in order to avoid major disturbances due to common mode tripping. In distribution networks however, the requirements are often quite different, e.g. instead of fault tolerance, rapid disconnection of DG is required in the event of network faults.
To be able to maintain power quality and reliability of a distribution network or a transmission network, the impact of wind power must be carefully studied. The interaction between a power plant and the power system is typically verified by means of power system simulations. In order to make these simulations possible, wind farm owners are required to provide system operators with the complete system models. Dynamic simulation using accurate system models is an indispensable tool both in transmission and distribution level interconnection studies.
THE IMPACT OF DISTRIBUTED GENERATION
Traditionally distribution networks have been designed to operate radially so that the power flows from upper voltage levels down to customers situated along the radial feeders. This has enabled a relatively straightforward protection strategy. When applying over-current protection, for example, it has been possible to assume that the fault current can have only one direction. However, this is now not always true if there are distributed generation (DG) units such as wind turbines in the network! As the share of distributed generation increases, distribution networks are becoming more like transmission networks where generation and load nodes are mixed, and a more complex protection system design is unavoidable.
In order to analyze the effects of distributed generation on the requirements for the protection of distribution networks, power system simulation studies are required. The dynamic modeling of various types of DG technologies such as wind energy is a necessity.
POTENTIAL PROBLEMS TO PROTECTION
Power system simulation and modeling studies have shown that distributed generation causes several challenges to the protection of distribution networks. The most commonly mentioned problems are the following:
- False tripping of feeders (sympathetic tripping)
- Nuisance tripping of production units
- Blinding of protection
- Increased or decreased fault levels
- Unwanted islanding
- Prohibition of automatic reclosing
- Unsynchronized reclosing
The appearance of these kinds of problems depends on both the characteristics of the network and DG. E.g., in short-circuit faults a wind power plant generates fault current that depends strongly both on the wind turbine generator type and the network configuration. Synchronous generators are able to feed rather large sustained fault current while inverter based systems may be controlled so that their output may be limited even to the rated current. From the point of view of protection coordination, the location of the fault in relation to generator and protection devices dictates the outcome of the fault situation.
Some critical problems related to DG are evaluated:
- The blinding of protection
- False tripping
- Anti-islanding protection
- Auto-reclosing
THE BLINDING OF PROTECTION - PREVENTING OVERCURRENT RELAY OPERATION
When a large distributed generation production unit or several small ones are connected to a medium voltage network, the fault current seen by the feeder protection relay may be reduced, which can lead to the prevention of the operation of overcurrent relays. This is also called protection under-reach.
Theoretically the problem can be described as follows. A situation, where a production generator unit is connected in a medium voltage (MV) feeder close to a primary substation, is studied. When a fault occurs at the end of the feeder the fault current consists of contributions both from the grid (I1) and from the generator (I2) as shown in Figure 1. The impedances involved in this situation are:
Zs = impedance of the grid and the primary transformer
Zg = impedance of the generator
ZL = impedance of the feeder (line)
Figure 1. Short-circuit fault in MV network and the corresponding single phase Thévenin equivalent circuit.
The current contribution from the distributed generator reduces the current seen by the feeder relay. We can conclude that the impact of the production unit increases with the size of the generator and with the length of the line section between the production unit and the fault.
Simulation Results
Figure 3 presents power system simulation results of the current seen by the feeder overcurrent relay, when a large (8 MW) synchronous generator (an example of distributed generation) is connected to different locations (0 km, 12 km, 20 km and 34 km) on the feeder, and a three-phase fault occurs at the end of the feeder. The relay pickup current is 300 A, and the time delay is 0.5 s. The fault current without the power plant would be ca. 420 A. The fault occurs at time 10.1 s. In three of the above mentioned cases, the power plant reduces the current seen by the protection so much that the relay will not start until the power plant trips, which in these cases takes several seconds.
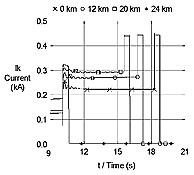
Figure 3. Current seen by the feeder overcurrent relay when the distance of a distributed power source from the substation is varied.
FALSE TRIPPINGS
The basic principle of false tripping is shown in Figure 4. The short-circuit fault occurs on feeder 2, but also feeder 1 is tripped because of overcurrent fed by the DG unit. False tripping (sympathetic tripping) is typically caused by synchronous generators, which are capable of feeding sustained short-circuit current.
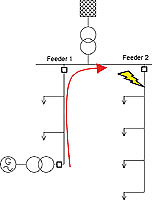
Figure 4. Principle of false tripping due to Distributed Generation.
In our study, false tripping of a healthy feeder system was verified by simulations with the 8 MW synchronous generator model. Figure 5 presents an example where a 3-phase short-circuit fault on an adjacent feeder causes the false tripping of feeder 1, because the power plant on feeder 1 feeds short-circuit current to the fault.

Figure 5. Example of false tripping caused by a short-circuit fault on adjacent feeder.
False tripping of healthy feeders can likely be solved by directional overcurrent relays, but with the following consideration: Protection against the bus faults may have to be changed. There should be a transfer trip from the main infeed relays (overcurrent relays on the secondary side of the primary transformer) and the arc protection relays to the feeder relays for the feeders having a significant amount of DG connected.
ANTI-ISLANDING PROTECTION
In the case of a sudden loss of grid connection, a part of the network may keep operating as an island. In most cases this is not desirable for the following reasons: • Reconnection of the islanded part becomes complicated, especially when automatic reclosing is used. This can lead to damage of equipment and can decrease the reliability of the network.
- The network operator is unable to guarantee the power quality in the island. There could be abnormal voltage levels or frequency fluctuations, and the fault level may be too low resulting in the overcurrent protection not working the way it is designed.
- Safety problems to maintenance personnel arise when de-energized circuits are back-fed.
In terms of achieving an adequate safety and reliability level in the distribution system, Anti-islanding protection is generally considered necessary. The rules and guidelines vary from country to country but requirements similar to the following are often given: • DG should be disconnected from the network in the case of an abnormality in voltage or frequency.
- If one or more phases is disconnected from the grid supply the DG should be rapidly disconnected from the network.
- If auto-reclosing is applied, the DG units must disconnect clearly before the reclosing, so that there will be enough time for the fault arc to extinguish.
Anti-islanding protection is very hard to accomplish by traditional means, e.g., the basic under-/over-voltage and under-/over-frequency relays may fail to operate if the power mismatch in the island that is created in the islanding situation is close to zero.
AUTORECLOSING
In overhead medium voltage networks, automatic reclosing is a very effective means to clear faults. In Finland for example, approximately 80% of faults can be cleared with high-speed autoreclosing and 15% with time-delayed autoreclosing. The impact of autoreclosing is based on the extinction of arc during the dead time of the reclosing sequence. In the Nordic countries of Europe, dead time is typically only 0.3 s.
Distributed generation seems to be rather incompatible with present reclosing practices. In suitable conditions, DG may prevent the arc extinction and the momentary fault can become a permanent fault. During the circuit breaker open time of the reclosing sequence, the generators in the network usually tend to drift away from the synchronism with respect to the grid. Thus the reconnection made without any synchronization, which is the usual way, may cause serious damages to the distributed generators, as well as high currents and voltages in the neighboring network.
Power system simulation studies have confirmed the high risk of out-of-phase reclosing. It can be safely stated that rapid and reliable loss-of-mains protection is needed. Distributed Generation units must be disconnected very rapidly during the breaker open time of an auto-reclosing sequence.
SUMMARY
According to the simulation studies performed with PSCAD, the proper coordination of the protection of network and distributed generation units is highly important in order to avoid false tripping of protection devices. In certain cases directional protection is also necessary. And, DG units that are capable of providing large short-circuit current may prevent the operation of feeder relays.
The primary results of these simulation studies have provided a clear indication of the potential protection problems that need to be solved by careful protection design or possibly through the introduction of new protection algorithms. Solutions are urgently needed since the percentage of distributed generation is increasing rapidly.
ACKNOWLEDGEMENT
The authors would like to acknowledge the insight and contributions of the following individual:
Dennis Woodford, President, Electranix Corporation for his contribution on the dynamic modeling of wind energy systems.
ABOUT THE AUTHORS
- Kimmo Kauhaniemi is a senior researcher at VTT Technical Research Centre of Finland and also a part-time Professor of Electrical Engineering at University of Vaasa, Finland.
- Lauri Kumpulainen is a researcher at VTT Technical Research Centre of Finland.
- Paul Buchanan is a Professional Engineer with the Manitoba HVDC Research Centre in Winnipeg, Canada. (www.hvdc.ca)
- Brahma, S.M., Girgis, A.A., 2002, IEEE PES Winter Meeting, Vol. 1, “Microprocessor-Based Reclosing to Coordinate Fuse and Recloser in a System with High Penetration of Distributed Generation”, 453 – 458
- Guillot, M., Collombet, C., Bertrand, P., Gotzig, B., 2001, CIRED, IEE Conf, Pub. No. 482, “Protection of Embedded Generation Connected to a Distribution Network and Loss of Mains Detection”
- Jenkins, N., Allan, R., Crossley, P., Kirschen, D., Strbac, G., 2000, Embedded Generation, IEE
- Redfern, M.A., Usta, O., Barrett, J.I., Fielding, G., 1993, 5th Int. Conf. on Developments in ower System Protection, “A new digital relay for loss of grid to protect embedded generation”, 127 -130
- DEFU, 1995, Relaebeskyttelse ved decentrale produktionsanlaeg med synkrongeneratorer. Teknisk rapport 293, 2. udgave
- Dugan, R.C., McDermott, T.E., 2002, IEEE Ind. Appl. Mag., Mar/Apr. 2002, 19 - 25
- Sobrink, K., Lovstrom Sorensen, P., ELTRA, Joncquel, E., EDF R&D, Woodford, D.A., Electranix Corp. Feasibility Study on Integration of LÆSØ SYD 160 MW Wind Farm using VSC Transmission", CIGRE SC14 Colloquium, Three Gorges Dam Site, 31 August, 2001. See also www.electranix.com/Papers/CIGRE-2001-VSCWind.pdf
- Matevosyan, J., Ackermann, T., Bolik, S., Söder, L., Comparison of International Regulations for Connection of Wind Turbines to the Network. Nordic Wind Power Conference – 2004: Grid Integration and Electrical Systems of Wind Turbines and Wind Farms. Chalmers University of Technology, Gothenburg, Sweden, 1-2 March 2004.